- What is ORP?
- Oxidation
- Reduction
- Oxidation-Reduction Potential (ORP) Analyzer Measuring Scale
- Understanding ORP and the Nernst Equation in Electrochemistry
- ORP Analyzer Working Principle
- Composition of ORP Measuring Electrode
- Composition of Reference Electrode
- Measurement Circuit of ORP Analyzer
- Applications of ORP Analyzer
- Advantages of ORP Analyzers
What is ORP?
The oxidation-reduction potential, often known as ORP, is a measurement that is expressed in millivolts and represents the likelihood that a chemical substance may either oxidize or reduce another chemical substance.
Oxidation
- Oxidation involves the loss of electrons from an atom, molecule, or ion, which can occur independently or alongside the addition of oxygen, from which the term originates.
- Common instances include the rusting of iron and the combustion of wood. Following oxidation, the oxidation state of the substance increases. Many substances can assume various oxidation states.
- For instance, sulfur can manifest oxidation states such as -2 (as in H2S), 0 (as in S), +4 (as in SO2), and +6 (as in SO4-2).
- Such substances can undergo sequential oxidation, transitioning from one oxidation state to the next higher one.
- Successive oxidation states of a specific substance constitute redox couples.
- In the example provided, the redox couple is Fe+2/Fe:
Fe = Fe+2 + 2e
- This expression represents the half-reaction for oxidation. However, as electrons released by the iron atom cannot persist in solution, they must be accepted by another substance in the solution.
- Thus, the complete reaction involving iron’s oxidation necessitates the presence of another substance, which undergoes reduction.
- Consequently, the oxidation reaction depicted for iron constitutes only half of the overall reaction that transpires.
Reduction
- Reduction entails the overall gain of electrons by an atom, molecule, or ion, leading to a decrease in its oxidation state.
- Similar to oxidation, substances capable of exhibiting multiple oxidation states can also undergo sequential reductions, transitioning from one oxidation state to the next lower one.
- The chemical equation presented below illustrates the half-reaction for the reduction of chlorine:
Cl2 + 2e- = 2Cl
- This represents the reduction of chlorine gas to chloride ions, with the redox couple identified as Cl2/Cl- (chlorine/chloride).
- Both reduction and oxidation take place concurrently in every redox process. Electrons lost during oxidation must find another substance as their destination, while electrons gained in reduction reactions must originate from a source.
- When combining two half-reactions to formulate the overall reaction, the number of electrons lost in the oxidation reaction must equal the number gained in the reduction reaction.
- In the provided example:
Oxidation: Fe = Fe+2 + 2 e–
(Half- Reaction)
Reduction: Cl2 + 2 e– = 2 Cl–
(Half- Reaction)
Overall Reaction: Fe + Cl2 => FeCl2
- In this reaction, iron (Fe) serves as the reductant or reducing agent, reducing chlorine (Cl2) to form iron chloride (FeCl2). Conversely, chlorine (Cl2) acts as the oxidizing agent, oxidizing iron (Fe).
Oxidation-Reduction Potential (ORP) Analyzer Measuring Scale
- The Oxidation-Reduction Potential (ORP) scale is a measure of a solution’s capacity for oxidation or reduction, given in millivolts.
- It quantifies the voltage difference between a measuring electrode and a reference electrode.
- Depending on the solution, ORP electrodes function either as electron donors or acceptors.
- Similar to pH, which gauges acidity or alkalinity based on hydrogen ion concentration, ORP reflects the overall electron activity within a solution.
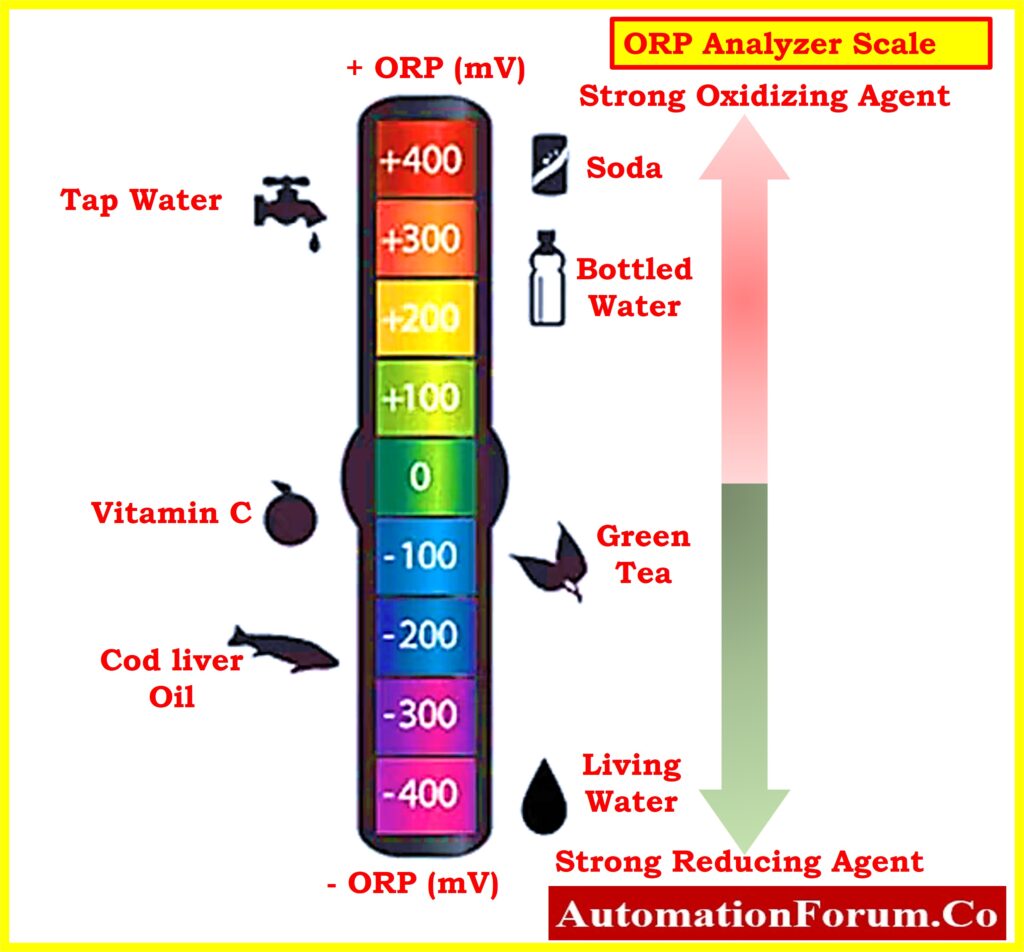
- The typical ORP scale spans from 1500 mV to -1500 mV, with 0 mV indicating neutrality.
- Solutions with ORP readings above 0 mV are deemed acidic, while those below 0 mV are considered alkaline.
- Unlike pH, which is primarily influenced by acids and bases, ORP values are affected by all oxidizing and reducing agents present in the solution.
- Under oxidizing conditions, the measuring probe loses electrons to the solution, resulting in a positive potential.
- Conversely, in reducing environments, electrons are donated to the probe, leading to a negative potential.
- Strong oxidizing agents, such as acid permanganate solutions, yield highly positive ORP values by avidly attracting electrons from the electrode.
- On the other hand, potent reducing agents, like sulfite solutions, generate strongly negative ORP values by forcefully pushing electrons into the electrode.
- While pH detects the amount of hydrogen ions precisely, ORP provides relative measurements of substances without making any distinctions between them.
- Despite its lack of specificity, ORP serves as a cost-effective method for monitoring various compounds such as chlorine, ozone, bromine, cyanide, and chromate, among others.
Understanding ORP and the Nernst Equation in Electrochemistry
Standard Potential
- The standard potential (denoted as E°) of a redox couple provides insight into how readily a substance can undergo oxidation or reduction.
- These potentials are referenced to the hydrogen ion/hydrogen (H+/H2) redox couple, with an assigned standard potential of 0 millivolts.
- Each redox couple’s standard potential, alongside its corresponding half-reaction, is typically cataloged in reference materials.
- In determining the Oxidation-Reduction Potential (ORP) of a solution, the Nernst equation proves invaluable.
- This equation considers the molar concentrations of the chemical substances involved in the half-reaction.
- By factoring in these concentrations, the equation calculates the ORP. The coefficient in the Nernst equation, derived from the number of electrons engaged in the half-reaction, dictates the magnitude of the logarithmic relationship between ORP and the concentrations.
Nernst Equation
In electrochemistry, the Nernst equation plays a crucial role in determining the equilibrium reduction potential of a half-cell within an electrochemical cell. Additionally, it aids in calculating the total voltage, also known as electromotive force, for a given system.
The Nernst equation is represented as:
Eh = E0 + (RT/nF) * log(Aox/Ared)
Where:
- Eh represents the Oxidation-Reduction Potential value of the reaction.
- E0 signifies the standard potential specific to the reaction series, which remains constant and is unaffected by factors such as Aox/Ared ratios or temperature.
- RT/nF is the Nernst number, incorporating the gas constant (R), temperature (T), the number of electrons transferred (n), and Faraday’s constant (F).
- Aox represents the activity of the oxidant.
- Ared denotes the activity of the reductant.
- For example, when examining hypochlorous acid (HOCl) in water, its ORP hinges on the concentrations of chloride ions (Cl-) and hydrogen ions (H+), in addition to the concentration of hypochlorous acid itself.
- The ORP exhibits a logarithmic correlation with these concentrations, with the coefficient derived from the number of electrons participating in the half-reaction.
- Notably, changes in concentration exert a proportional influence on ORP, as reflected by the coefficient in the Nernst equation. Unlike pH, ORP measurements typically lack temperature compensation due to the diverse effects of temperature on ORP reactions.
- When assessing the impact of individual substances in the half-reaction, the Nernst equation facilitates the calculation of their contributions based on their anticipated concentration ranges.
ORP Analyzer Working Principle
The process of measuring Oxidation-Reduction Potential (ORP) involves the use of an ORP sensor, which comprises an ORP electrode and a reference electrode, similar to the setup for pH measurement.
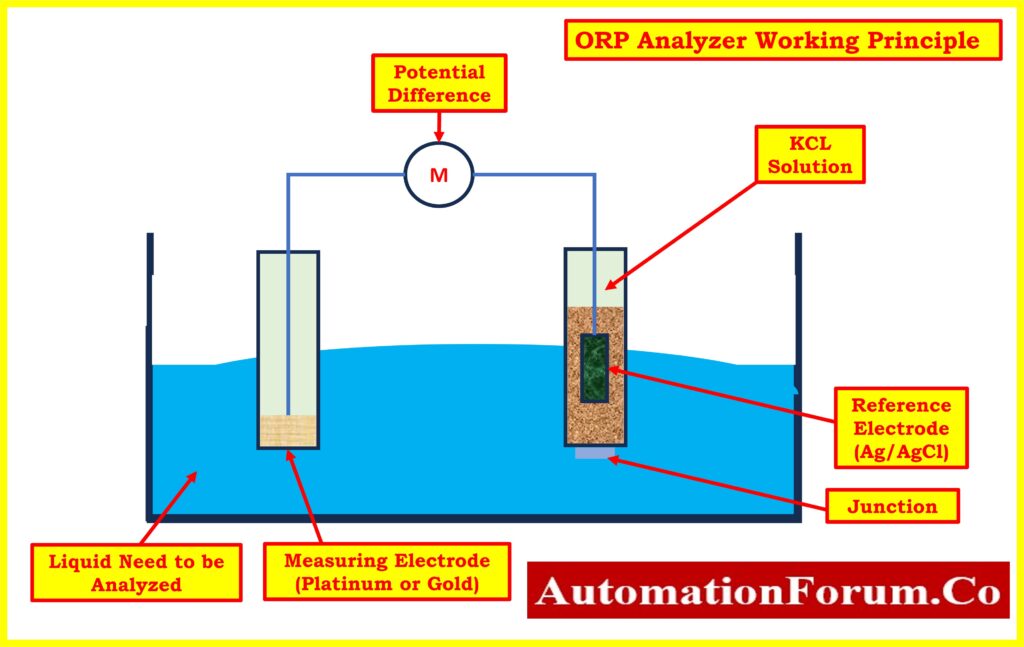
Composition of ORP Measuring Electrode
- The ORP electrode operates based on the principle of utilizing an inert metal electrode, typically platinum or occasionally gold, due to its low resistance.
- This electrode either releases electrons to an oxidant or accepts electrons from a reductant present in the solution.
- As electron exchange occurs, the ORP electrode develops a potential equal to the ORP of the solution.
- The typical accuracy of ORP measurement is within ±5 mV. However, in certain instances, electron exchange may be hindered by a low rate of electron exchange, leading to potential measurement errors.
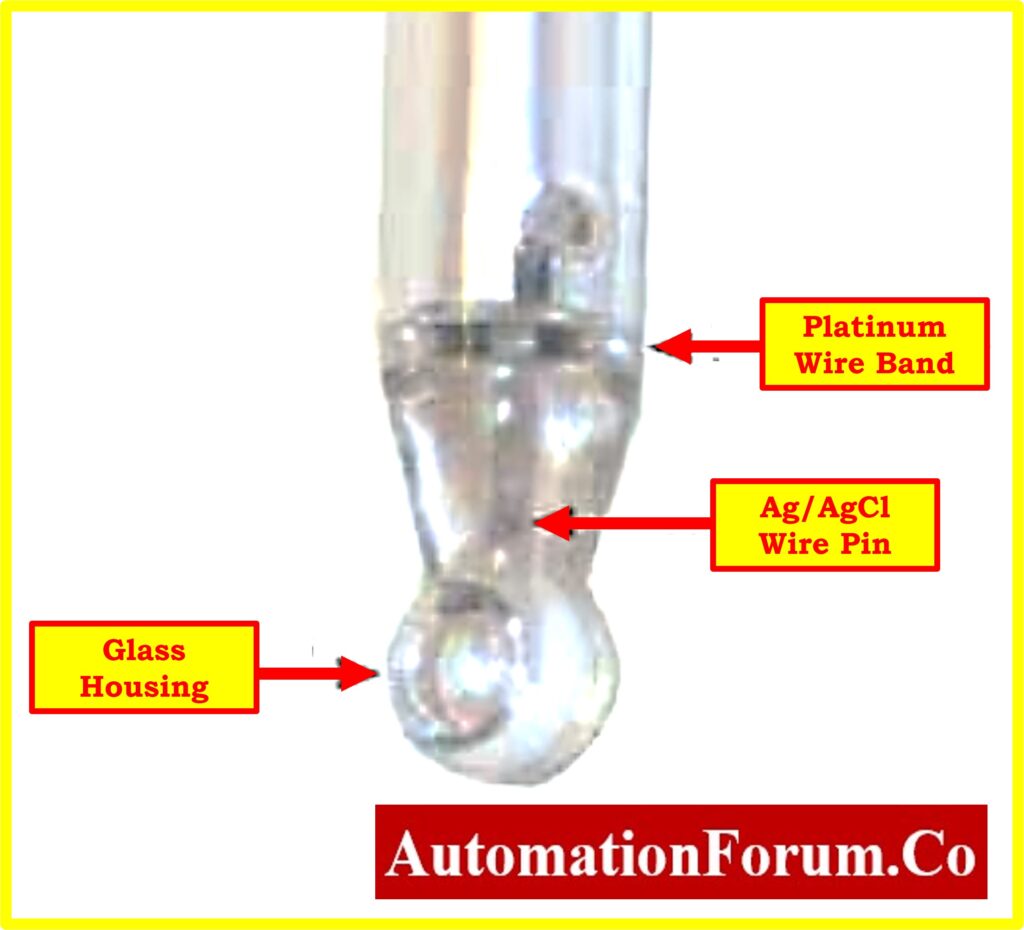
Composition of Reference Electrode
- For ORP measurements, the reference electrode used is typically the same silver-silver chloride electrode utilized in pH measurements.
- Unlike in pH measurements, some deviation in the reference electrode’s performance is acceptable for ORP measurements, as the millivolt changes observed in most ORP applications tend to be significant.
- In specific applications, such as bleach production, alternative reference electrodes, such as a silver billet or a pH electrode, may be employed based on process requirements.
Measurement Circuit of ORP Analyzer
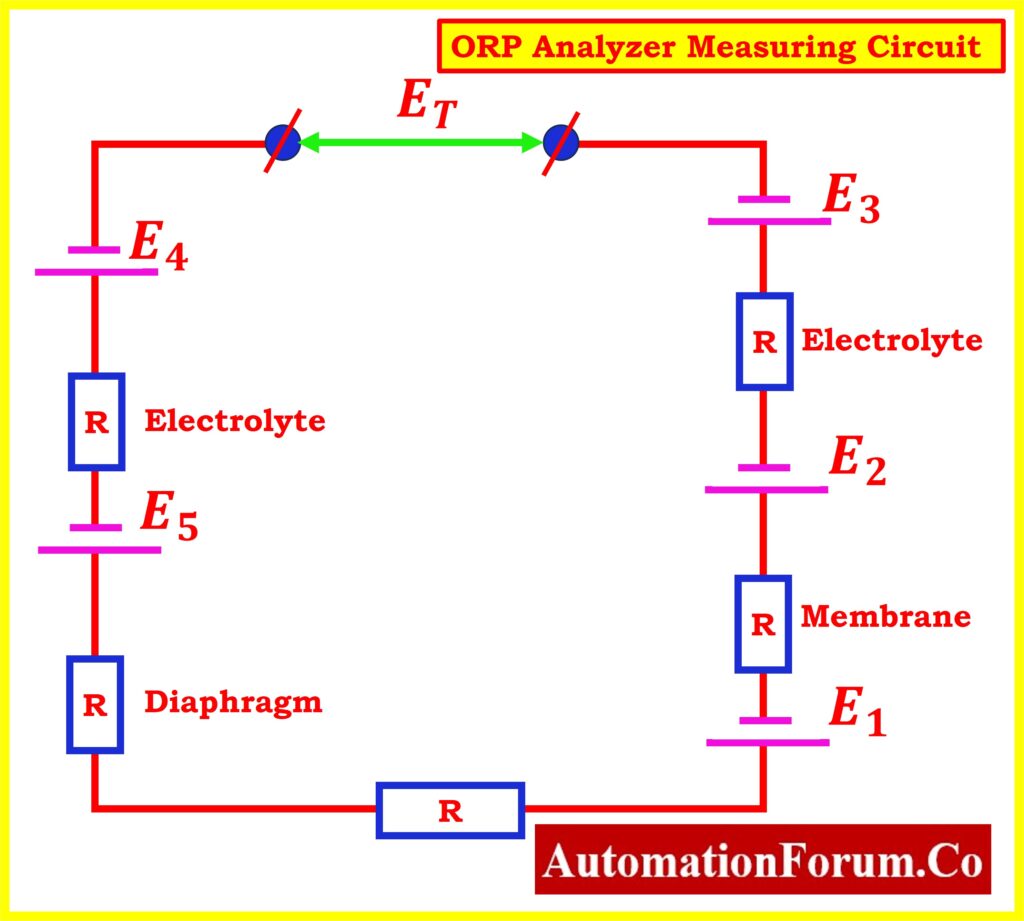
Similar to a millivolt meter, an ORP probe measures the small voltage differences that exist between a process solution-filled reference electrode (the negative terminal) and a platinum measuring electrode (the positive terminal).
- The voltage differential between these electrodes is measured by the analyzer.
- These voltage fluctuations provide insight into the oxidizing or reducing potential of substances within a solution.
- Response time varies based on the concentration of the redox system; higher concentrations yield quicker responses, while lower concentrations result in slower ones.
- Within the measurement circuit, several potentials hold significance:
E1: The potential that exists between the surface of the ORP metal and the process
E2: Potential between the reference electrode and the electrolyte
E3: Potential developed at the surface of the electrolyte and the process
- The signal converter calculates the total of these potential differences:
Et = E1 + E2 + E3
- It’s vital that the analyzer employed for detection features high impedance (resistance) to accurately measure the minute voltages or charge accumulation generated by the continuous acceptance and release of electrons on the ORP electrode.
- While achieving ideal conditions may not always be feasible, a small potential difference in the reference is generally acceptable since most millivolt changes measured for ORP within solutions tend to be substantial.
Applications of ORP Analyzer
ORP finds numerous applications in monitoring and controlling oxidation-reduction reactions across various industries:
- Cyanide Destruction: ORP is utilized to monitor and control processes involved in the destruction of cyanide compounds.
- Dechlorination: It is employed in dechlorination processes to ensure the effective removal of chlorine compounds from water or other solutions.
- Chromate Reduction: ORP is utilized in processes aimed at reducing chromate compounds to less harmful forms.
- Hypochlorite Bleach Production: ORP is crucial in the production of hypochlorite bleach, ensuring the optimal conditions for its manufacture.
- Scrubber Monitoring: Monitoring of chlorine and chlorine dioxide scrubbers using bisulfite involves ORP measurements to maintain efficient scrubbing operations.
- Leak Detection: ORP can serve in leak detection by detecting the presence of oxidants or reductants in certain cases.
- Biological Growth Control: ORP is employed in controlling biological growth by maintaining a minimum ORP value, which effectively destroys microorganisms. This approach is utilized in chlorination processes for swimming pools and cooling towers. Notably, pH control is also integral to these applications.
Advantages of ORP Analyzers
- ORP analyzers provide real-time measurements of oxidation-reduction potential, enabling prompt adjustments to process conditions.
- By monitoring ORP levels, processes involving oxidation-reduction reactions can be effectively controlled and optimized for efficiency and quality.
- ORP analyzers are versatile and can be used in a wide range of applications, including water treatment, chemical processing, and industrial manufacturing.
- ORP measurements can help detect potential issues such as contamination, leaks, or process inefficiencies before they escalate, allowing for timely corrective actions.
- ORP analyzers are valuable tools for environmental monitoring, allowing for the assessment of water quality and the detection of pollutants or contaminants.
FAQ
What is the use of ORP analyzer?
ORP analyzers measure oxidation-reduction potential in solutions, aiding in monitoring and controlling various processes like cyanide destruction, water dechlorination, chromate reduction, and bleach production across industries.
What is a good ORP level?
A good ORP level varies depending on the application, but generally, for water disinfection, levels between +600 mV to +800 mV are considered effective in controlling biological growth and ensuring water quality.
What is the difference between TDS and ORP?
TDS (Total Dissolved Solids) measures the concentration of dissolved substances, including minerals, salts, and metals, in water. ORP (Oxidation-Reduction Potential) gauges the ability of a solution to oxidize or reduce substances. pH indicates the acidity or alkalinity of water.
What is the unit of ORP?
ORP (Oxidation-Reduction Potential) is indeed measured in millivolts (mV) using an ORP meter. Positive ORP readings signify oxidizing agents, with higher values indicating greater oxidation potential. Conversely, negative ORP readings suggest reducing agents.